Understanding the Microbiota
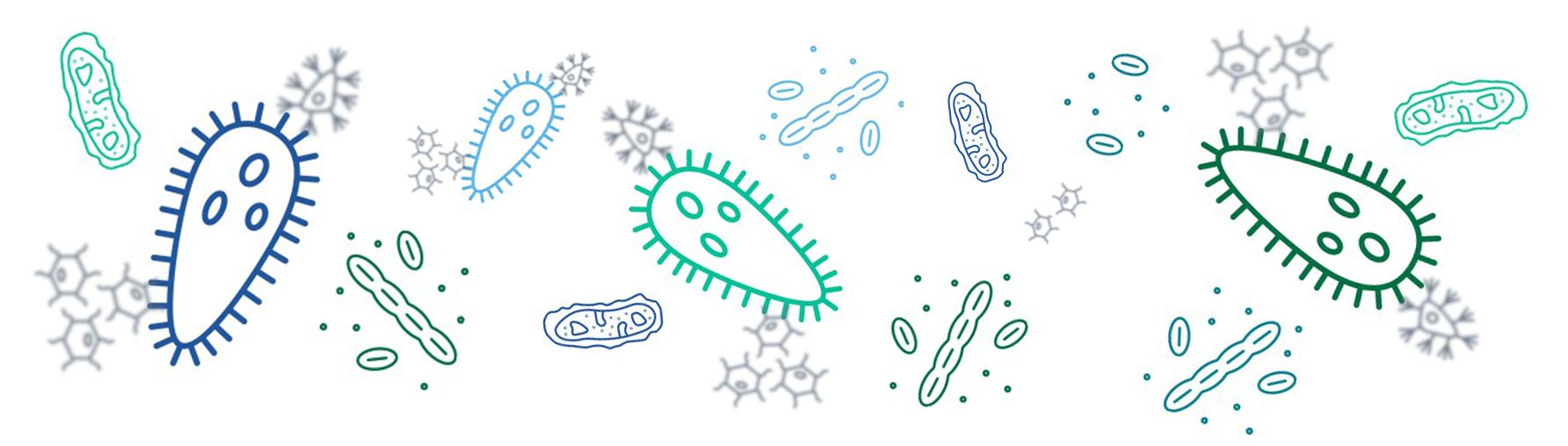
What is the microbiota?
Thousands of years of coexistence have given rise to intricate relationships forming between animals and the microbes which live on or within them. This consortium of micro-organisms is made up mainly of bacteria, however 2% of the microbial DNA has been isolated from fungi,1 and protozoa, archaea and viruses are also known to be present. Together these micro-organisms form complex ecosystems, on or within the body, known as microbiota. While microbes have created ecosystems all over our bodies, we shall focus on the gastrointestinal (GI) microbiota, as this has been the subject of most research. There are estimated to be as many bacterial cells as there are human cells within the body,2 with the combined microbial genetic pool outnumbering the host gene content by a multiple of 100.3 Until fairly recently, bacterial culture was the only available method to identify bacterial species present within the intestinal microbiota. Given that only 20 percent of the intestinal bacteria are culturable,3 the numbers and species identified were hugely underestimated. The use of PCR amplification of 16S rRNA genes has enabled a more accurate insight into the bacterial populations present in the microbiota, and provides a way to investigate the microbial function via its gene content, known as the microbiome. Using this method, more than 2000 species of bacteria have been isolated from humans.4
The composition of the GI microbiota varies between individuals and between specific compartments of the gastrointestinal tract (GIT) within an individual.5 However, despite differing presence and abundance of specific microbes, it has been shown in dogs6 and people7 that the functional genetic content of the microbiota is conserved across individuals.
How does the microbiota become established?
Traditionally it was thought that the foetus developed in a sterile environment in the womb, with the first bacterial exposure occurring during birth. However, studies have demonstrated the presence of bacterial DNA in the placenta and amniotic fluid from healthy term pregnancies, not thought to be associated with intra-amniotic infection, and umbilical cord blood from neonates delivered by caesarean.8,9 Further to this, it has been shown that genetically labelled Enterococcus faecium given to pregnant mice, is found in the meconium of their pups, despite delivery by caesarean.10 Whilst live bacteria have not yet been identified, this does suggest some degree of in utero colonisation.
As they pass through the mother’s birth canal, infants are covered in vaginal and faecal microbes which contribute to the establishment of skin, oral, nasopharyngeal and gut microbiota. These infants acquire microbiota similar to that of the vagina, whereas infants born via caesarean develop a microbiota more similar to human skin.11 These differences in microbiota may result in long term health consequences to the child, in particular concerning immune-mediated disease. For example, children born via C-section are significantly more likely to develop allergic rhinitis, asthma, celiac disease, type 1 diabetes, and inflammatory bowel disease.12-15
Maternal microbial transmission continues beyond birth via breastfeeding. The mother’s milk contains hundreds of bacterial species16. Amongst some of the earliest species to be transmitted to the infant are Bifidobacterium longum subsp. infantis, which has been shown to have adaptations to promote milk utilisation.17
As the infant approaches weaning, the composition of the microbiota shifts to favour plant digestion. Unsurprisingly, introduction of varying food sources results in large changes to the microbiota.18 As dietary and environmental changes settle after the first few years of life, the established microbiota becomes fairly stable for that individual.19 Alterations may then occur following illness or infection, environmental or dietary change or exposure to certain medications, such as antibiotics.20,21
Since the blue-print of an individual’s microbiota is established in the first few years of life, alterations to the microbial populations during this time can have life-long effects on the host. For example administration of antibiotics early in life can alter the microbiota, which has been linked to an increased risk of obesity and allergies.21-23 It is proposed that excessive hygiene in childhood can affect the development of the microbiota and result in increased risk of allergies and inflammatory bowel disease later in life.24-26 This is known as the hygiene hypothesis.
What does the microbiota do and why do we need it?
We have discussed what the microbiota is and how it is established, but why is it so important?
A balanced microbiota is essential for host health in the following ways.
A healthy microbiota:
- promotes normal gastrointestinal development. This is shown through the following examples, where the absence of a microbiota results in structural changes in the gastrointestinal tract. Antibiotic administration to rats at the end of gestation resulted in pups with small stomachs, reduced acid secretion and increased intestinal permeability.27 Germ free mice have been shown to have poor capillary development in the intestinal villi; however, this can be reversed by faecal microbial transplantation from mice with normal microbiota.28 Antibiotics given to pregnant sows resulted in altered intestinal architecture in their piglets.29
- is involved in immune regulation. One of the most important roles of the microbiota is in the development of oral tolerance – the suppression of immune responses to harmless orally ingested antigens, and commensal bacteria. The dendritic cells present in the intestinal mucosa act to detect luminal contents including commensal bacteria, since these are harmless they stimulate creation of an anti-inflammatory environment.30
- helps in defence against pathogenic organisms. This is not only by its close interaction with the immune system. The commensal bacteria also compete with pathogens for food sources and epithelial binding sites, and promote mucus production which creates a barrier between pathogenic bacteria and the gut epithelium.31
- provides beneficial nutritional effects. By fermenting dietary substances, which are indigestible to the host, short-chain fatty acids are produced. These have many host benefits such as providing vital nutrition for enterocytes, modulating immune function and increasing mucosal blood flow.32,33
- is involved in bile acid metabolism. Only gut bacteria can convert primary bile acids to secondary bile acids, the ratio between these is important in gut homeostasis. Alterations to bile acid metabolism may have negative health consequences. 34,35
- is involved in the synthesis of certain vitamins. Gut microbiota can synthesize certain vitamins, notably Vitamin K and B group vitamins.36
What is dysbiosis?
Broadly defined, dysbiosis is any change to the composition of resident commensal microbial communities relative to the community found in healthy individuals.37 There may be a change in the species present or the abundance of each species present. Dysbiosis can be deleterious to the host as there may be an increase in pathogenic bacteria, which can be directly damaging to the host; or there may be a reduction in commensal bacteria, disrupting the normal functions of the microbiota as explained above. Dysbiosis may result in immune dysregulation, including reduced anti-inflammatory metabolites, and abnormal metabolism of dietary carbohydrates, bile acids and vitamins.
Dysbiosis has been described in dogs with inflammatory bowel disease (IBD) and acute diarrhoea, cats with chronic enteropathies, dogs and cats with Giardia duodenalis and dogs with meningoencephalomyelitis of unknown origin (MUO).38-45 In people dysbiosis is associated with a huge number of disease conditions, a few examples include: diarrhoea, IBD, IBS, obesity, colorectal cancer and diabetes.46-53
The involvement of the microbiota and dysbiosis in obesity is a hot-topic for research. One interesting study demonstrated that giving the microbiota from obese mice to germ free mice resulted in greater weight gain than when the microbiota from lean mice was given to germ free mice, despite being fed the same diet. On assessment of the microbiome, increased genes involved in energy extraction were found in the faeces from the obese mice.54
What are probiotics?
Probiotics help to support a healthy gut microbiomeand are defined by the World Health Organisation as “live microorganisms which when administered in adequate amounts confer a health benefit on the host”.55
What to check when looking for a probiotic:
A high quality probiotic product should state:
- The organism(s) present, right down to the strain
- The numbers of colonies present. (WHO recommends >1 x 109 CFU/kg)
- The date by which the bacteria are guaranteed to be present at that level
References
- Swanson KS, Dowd SE, Suchodolski JS, et al. Phylogenetic and gene-centric metagenomics of the canine intestinal microbiome reveals similarities with humans and mice. ISME J 2011; 5: 639–649.
- Sender R, Fuchs S, Milo R. Revised estimates for the number of human and bacteria cells in the body. PLoS Biol 2016; 14(8)
- Suchodolski JS. Diagnosis and interpretation of intestinal dysbiosis in dogs and cats. Vet J 2016; 215: 30-37.
- Hugon P, Dufour JC, Colson P, Fournier PE, Sallah K, Raoult D. A comprehensive repertoire of prokaryotic species identified in human beings. Lancet Infect Dis 2015; 15(10): 1211-1219.
- Suchodolski JS, Ruaux CG, Steiner JM, Fetz K, Williams DA. Assessment of the qualitative variation in bacterial microflora among compartments of the intestinal tract of dogs by use of a molecular fingerprinting technique. Am J Vet Res 2005; 66(9): 1556-1562
- Guard BC, Suchodolski JS. Canine intestinal microbiology and metagenomics: From phylogeny to function. J Anim Sci 2016; 94(6): 2247-2261.
- The Human Microbiome Project Consortium. Structure , function and diversity of the healthy human microbiome. Nature 2012; 486: 207-214.
- Steel JH, Malatos S, Kennea N, et al. Bacteria and inflammatory cells in fetal membranes do not always cause preterm labor. Pediatr Res 2005; 57: 404–411.
- Jiménez E, Fernández L, Marín ML, et al. Isolation of commensal bacteria from umbilical cord blood of healthy neonates born by cesarean section. Curr Microbiol 2005; 51: 270–274.
- Jiménez E, Marín ML, Martín R, et al. Is meconium from healthy newborn actually sterile? Res Microbiol 2008; 159: 187–193.
- Dominguez-Bello MG, Costello EK, Contreras M et al. Delivery mode shapes the acquisition and structure of the initial microbiota across multiple body habitats in newborns. Proc Natl Acad Sci U S A 2010; 107: 11971–11975.
- Renz-Polster H, David MR, Buist AS et al. Caesarean section delivery and the risk of allergic disorders in childhood. Clin Exp Allergy 2005; 35(11): 1466–1472.
- Decker E, Engelmann G, Findeisen A et al. Cesarean delivery is associated with celiac disease but not inflammatory bowel disease in children. Pediatrics 2010; 125(6): 1433–1440.
- Cardwell CR, Stene LC, Joner G et al. Caesarean section is associated with an increased risk of childhood on set type 1 diabetes mellitus: a meta-analysis of observational studies. Diabetologia 2008; 51(5): 726–735.
- Bager P, Simonsen J, Nielsen NM, Frisch M. Cesarean section and offspring’s risk of inflammatory bowel disease: a national cohort study. Inflamm Bowel Dis 2012; 18(5): 857–862.
- Cabrera-Rubio R, Collado MC, Laitinen K, Salminen S, Isolauri E, Mira A. The human milk microbiome changes over lactation and is shaped by maternal weight and mode of delivery. Am J Clin Nutr 2012; 96: 544–551.
- Sela DA, Chapman J, Adeuya A et al. The genome sequence of Bifidobacterium longum subsp. infantis reveals adaptations for milk utilization within the infant microbiome. Proc Natl Acad Sci USA 2008; 105: 18964–18969.
- Koenig JE, Spor A, Scalfone N et al. Succession of microbial consortia in the developing infant gut microbiome. Proc Natl Acad Sci U S A 2011; 108: 4578-4585.
- Faith JJ, Guruge JL, Charbonneau M et al. The long-term stability of the human gut microbiota. Science 2013; 341(6141): 1237439
- David, LA, Maurice CF, Carmody RN et al. (2014) Diet rapidly and reproducibly alters the human gut microbiome. Nature 505(7484): 559–563.
- Cho I, Yamanishi S, Cox L et al. Antibiotics in early life alter the murine colonic microbiome and adiposity. Nature 2012; 488(7413), 621–626.
- Saari A, Virta LJ, Sankilampi U, Dunkel L, Saxen H. Antibiotic exposure in infancy and risk of being overweight in the first 24 months of life. Pediatrics 2015; 135(4): 617–626.
- Metsala J, Lundqvist A, Virta LJ, Kaila M, Gissler M, Virtanen SM. 2015. Prenatal and post-natal exposure to antibiotics and risk of asthma in childhood. Clin Exp Allergy 2015; 45(1), 137–145.
- Wold AE. The hygiene hypothesis revised: is the rising frequency of allergy due to changes in the intestinal flora? Allergy 1998; 53 (46): 20–25.
- Yazdanbakhsh M, Kremsner PG, van Ree R. Allergy, parasites, and the hygiene hypothesis. Science 2002; 296(5567), 490–494.
- Gent AE, Hellier MD, Grace RH, Swarbrick ET, Coggon D. Inflammatory bowel disease and domestic hygiene in infancy. Lancet 1994; 343(8900), 766–767.
- Fåk F, Ahrné S, Molin G, Jeppsson B, Weström B. Microbial manipulation of the rat dam changes bacterial colonization and alters properties of the gut in her offspring. Am J Physiol Gastrointest Liver Physiol 2008; 294(1): G148–154.
- Stappenbeck TS, Hooper LV, Gordon JI. Developmental regulation of intestinal angiogenesis by indigenous microbes via Paneth cells. Proc Natl Acad Sci U S A 2002; 99(24): 15451–15455
- Arnal ME, Zhang J, Messori S, Bosi P, Smidt H, Lallès JP. Early changes in microbial colonization selectively modulate intestinal enzymes, but not inducible heat shock proteins in young adult swine. PLoS One 2014; 9(2): e87967.
- Chistiakov DA, Bobryshev YV, Kozarov E, Sobenin IA, Orekhov AN. Intestinal mucosal tolerance and impact of gut microbiota to mucosal tolerance. Front Microbiol 2014; 5: 781.
- Kamada N, Chen GY, Inohara N, Núñez G. Control of Pathogens and Pathobionts by the Gut Microbiota. Nat Immunol 2013; 14(7): 685–690.
- Savage, D.C. Gastrointestinal microflora in mammalian nutrition. Annu Rev Nutr 1986; 6, 155–178.
- Marteau P, Seksik P, Lepage P, Doré J. Cellular and physiological effects of probiotics and prebiotics. Mini Rev Med Chem 2004; 4(8): 889–896.
- Pavlidis P, Powell N, Vincent RP, Ehrlich D, Bjarnason I, Hayee B. Bile acids and intestinal inflammation-luminal aggressors or regulators of mucosal defence? Aliment Pharmacol Ther 2015; 42, 802–817.
- Duboc H, Rajca S, Rainteau D. Connecting dysbiosis, bile-acid dysmetabolism and gut inflammation in inflammatory bowel diseases. Gut 2013; 62(4): 531–539.
- Hill MJ. Intestinal flora and endogenous vitamin synthesis. Eur J Cancer Prev 1997; 6(1): S43-45.
- Petersen C, Round JL. Defining dysbiosis and its influence on host immunity and disease. Cell Microbiol 2014; 16(7): 1024–1033.
- Suchodolski JS, Xenoulis PG, Paddock CG, Steiner JM, Jergens AE. Molecular analysis of the bacterial microbiota in duodenal biopsies from dogs with idiopathic inflammatory bowel disease. Vet Microbiol 2010; 142(3-4): 394-400.
- Suchodolski JS, Markel ME, Garcia-Mazcorro JF et al. 2012. The fecal microbiome in dogs with acute diarrhea and idiopathic inflammatory bowel disease. PLoS ONE 2012; 7(12), e51907.
- Guard BC, Barr JW, Reddivari L et al. Characterization of microbial dysbiosis and metabolomic changes in dogs with acute diarrhea. PLoS ONE 2015; 10(5), e0127259.
- Minamoto Y, Otoni CC, Steelman SM et al. Alteration of the fecal microbiota and serum metabolite profiles in dogs with idiopathic inflammatory bowel disease. Gut Microbes 2015; 6(1), 33–47.
- Xenoulis PG, Palculict B, Allenspach K, Steiner JM, Van House AM, Suchodolski JS. Molecular- phylogenetic characterization of microbial communities imbalances in the small intestine of dogs with inflammatory bowel disease. FEMS Microbiol Ecol 2008; 66(3), 579–589.
- Suchodolski JS, Foster ML, Sohail MU et al. The fecal microbiome in cats with diarrhea. PLoS ONE 2015; 10: e0127378
- Slapeta J, Dowd SE, Alanazi AD, Westman ME, Brown GK. Differences in the faecal microbiome of non-diarrhoeic clinically healthy dogs and cats associated with Giardia duodenalis infection: Impact of hookworms and coccidia. Int J Parasitol 2015; 45(9-10): 585–594.
- Jeffery ND, Barker AK, Alcott CJ et al. The Association of Specific Constituents of the Fecal Microbiota with Immune-Mediated Brain Disease in Dogs. PLoS One 2017; 12(1): e0170589.
- Pop M, Walker AW, Paulson J et al. Diarrhea in young children from low-income countries leads to large-scale alterations in intestinal microbiota composition. Genome Biol 2014. 15(6): R76
- Morgan XC, Tickle TL, Sokol H et al Dysfunction of the intestinal microbiome in Inflammatory bowel disease and treatment. Genome Biol 2012. 13(9): R79
- Qin J, Li R, Raes J et al. A human gut microbial gene catalogue established by metagenomics sequencing. Nature 2010; 464: 59–65.
- Jalanka-Tuovinen J, Salojärvi J, Salonen A et al. Faecal microbiota composition and host–microbe cross-talk following gastroenteritis and in post-infectious irritable bowel syndrome. Gut 2014; 63(11): 1737–1745.
- Perry RJ, Peng L, Barry NA et al. Acetate mediates a microbiome–brain–b cell Axis to promote metabolic syndrome. Nature 2016; 534(7606): 213–217.
- Ley RE, Bäckhed F, Turnbaugh P, Lozupone CA, Knight RD, Gordon JI. Obesity alters gut microbial ecology. Proc Natl Acad Sci USA 2005; 102(31):11070–11075.
- R. Gao, Z. Gao, L. Huang, and H. Qin. Gut microbiota and colorectal cancer. Eur J Clin Microbiol Infect Dis 2017; 36(5): 757–769.
- Gülden E, Wong S, Wen L. (2015) The gut microbiota and Type1 Diabetes. Clin Immunol 2015; 159(2): 143–153
- Turnbaugh PJ, Ley RE, Mahowald MA, Magrini V, Mardis ER, Gordon JI. An obesity-associated gut microbiome with increased capacity for energy harvest. Nature 2006; 444(7122): 1027–31.
- Guidelines for the Evaluation of Probiotics in Food. FAO/WHO (2002) click here for pdf (accessed February 7, 2019)